Environmental Robotics and Self-sustainable Technologies
WiRES – Waste into Resources, Energy and Sensing
Background
Our mission is to develop and implement advanced environmental technologies that operate autonomously to minimise ecological impact. By transforming waste into valuable resources, energy, and sensing capabilities, we aim to create self-sustaining systems that contribute to a cleaner, greener, and more sustainable future for all.
Located in the Bristol Robotics Laboratory (BRL) and representing University of the West of England (UWE) we are dedicated to reducing carbon footprint and promoting sustainable practices through research, community engagement and education.
Research Group: WiRES – Waste into Resources, Energy and Sensing
Why Environmental Robotics and Self-sustainable Technologies?
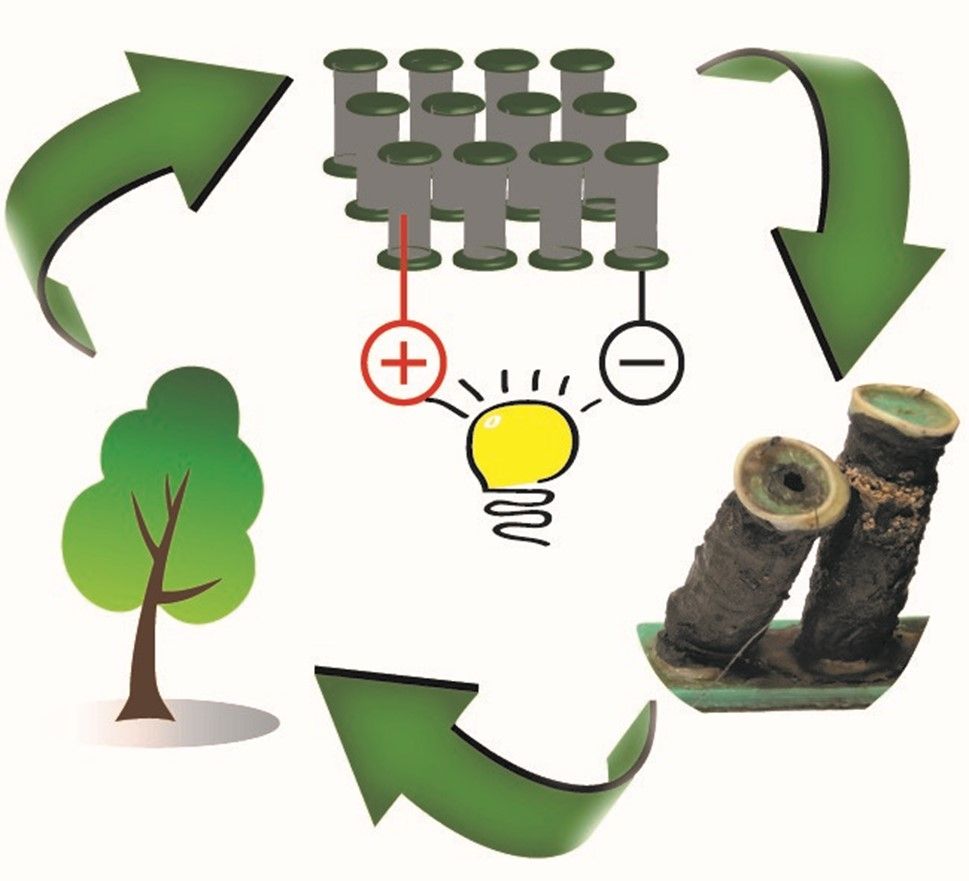
Environmental robotics and self-sustainable technologies offer innovative solutions for addressing and mitigating environmental challenges. Our research integrates microbiology, environmental science, electrochemistry and engineering to develop cutting-edge bioelectrochemical systems (BES). These systems leverage the unique capabilities of microbial metabolism, interacting with electrodes in fuel cell configurations, to create autonomous systems that can operate in diverse and demanding environments.
This interdisciplinary approach not only enhances our ability to monitor environmental conditions but also provides sustainable solutions to ecological issues. By integrating these fields, we aim to pioneer technologies that are both effective and environmentally friendly, making a tangible impact on global sustainability.
At the heart of our research are bioelectrochemical systems (BES), such as microbial fuel cells (MFCs). These systems harness the ability of electroactive microorganisms to convert organic matter into electrical energy to power robots.
In the context of environmental robotics, BES serve three main functions:
- Power Generation: MFCs can provide a sustainable power source for robotic systems, enabling long-term operation in remote or challenging environments
- Environmental Sensing: The microbial activity in BES can be used to detect and monitor environmental parameters, such as water quality or pollutant levels
- Pollution Cleanup and Waste Treatment: microbes incorporated in BES technologies contribute to more efficient pollutant removal.
Applications of Environmental Robotics
Biodegradable power sources
The concept of "Fade to green" involves using biodegradable stacks of MFCs, which harness the metabolic processes of microbes to generate electricity. This approach aligns with the lifecycle of soft robots that can eat, drink, live, die, and decay, integrating seamlessly into natural environments. Studies comparing the stability of biodegradable, ceramic, and cation exchange membranes in MFCs highlight the potential for long-term use, with biodegradable options offering a sustainable alternative that reduces environmental impact while maintaining efficiency.
- Fade to green: a biodegradable stack of microbial fuel cells
- Eating, drinking, living, dying and decaying soft robots
- Comparing the short and long term stability of biodegradable, ceramic and cation exchange membranes in microbial fuel cells
Environmental Monitoring - Sensing
Robots equipped with BES can be deployed for continuous water quality monitoring in various aquatic environments. Sensing using microbial fuel cells (MFCs) has shown great potential in environmental monitoring and energy harvesting. The long-term feasibility study of in-field floating MFCs demonstrated their effectiveness in monitoring anoxic wastewater while generating power, proved stable and reliable, making them suitable for remote sensing applications. Additionally, research on the stability and reliability of anodic biofilms under different feedstock conditions highlighted the adaptability of MFC sensors. By maintaining stable power output and biofilm activity, these sensors can effectively monitor environmental changes, providing a sustainable and efficient solution for real-time data collection.
- Long term feasibility study of in-field floating microbial fuel cells for monitoring anoxic wastewater and energy harvesting
- Stability and reliability of anodic biofilms under different feedstock conditions: Towards microbial fuel cell sensors
Autonomous Resource Recovery
Autonomous recovery in the context of resources involves harnessing the microbial power to convert waste into valuable resources, such as nutrients and clean water, which can be reused, for example in agriculture. By integrating BES, this approach not only enhances resource efficiency but also promotes sustainability by reducing waste and minimising the reliance on chemical fertilisers.
MFCs have shown nutrient recovery and electricity generation in innovative ways including dual benefits of electricity generation and the recovery of struvite, a valuable fertiliser, while producing power. Through recovery, showing how MFCs can enhance wastewater treatment by recovering key macronutrients and water as a by-product. These advancements highlight the potential of MFCs in creating sustainable and efficient systems for nutrient recovery and environmental protection.
- Electricity generation and struvite recovery from human urine using microbial fuel cells
- Water formation at the cathode and sodium recovery using microbial fuel cells (MFCs)
- Microbial Fuel Cell-driven caustic potash production from wastewater for carbon sequestration
Disinfectant production and electrosynthesis of catholyte
MFC demonstrate the electrosynthesis of catholyte, a disinfectant, during electricity generation from wastewater, highlighting the dual benefits of power production and sanitation. The catholyte formation at the cathode is showing how MFCs can enhance wastewater treatment by recovering valuable resources (nutrients and water). Additionally, MFCs have been used to generate disinfectant from urine through electroosmotic drag, effectively inactivating pathogenic species such as Salmonella. The improved electrofiltration and long-term power output is making these systems more efficient and sustainable.
- Electroosmotically generated disinfectant from urine as a by-product of electricity in microbial fuel cell for the inactivation of pathogenic species
- Electricity and disinfectant production from wastewater: Microbial Fuel Cell as a self-powered electrolyser
- Simultaneous electricity generation and microbially-assisted electrosynthesis in ceramic MFCs
- A new method for urine electrofiltration and long term power enhancement using surface modified anodes with activated carbon in ceramic microbial fuel cells
Sanitation
Implementation of MFCs in the field of sanitation is offering a dual solution to wastewater treatment and energy generation. This technology not only improves sanitation in remote and off-grid areas but also provides a renewable energy source for lighting and other small electrical devices. The success of MFCs in treating urine and generating power opens up new possibilities for sustainable sanitation solutions, particularly in regions lacking proper infrastructure. Field Trials included:
Carbon Capture
Carbon capture can be achieved by electro-osmotic-based catholyte production, where MFCs generate a caustic catholyte that captures carbon dioxide by forming carbonate and bicarbonate salts. This process not only aids in carbon capture but also enhances the environmental sustainability of wastewater treatment. Additionally, MFCs can produce self-sustainable electricity from algae, leveraging the photosynthetic capabilities of algae to generate oxygen and power simultaneously. This method integrates wastewater treatment with energy production, creating a closed-loop system that maximises resource efficiency and sustainability.
Living Architecture
Living architecture powered by MFCs represents an innovative approach to creating energy-generating buildings. This concept integrates MFC technology directly into building materials, such as bricks, to produce electricity from organic matter while simultaneously treating waste. The technology's potential extends beyond electricity generation, as it can also contribute to wastewater treatment and reduce the environmental impact of buildings, marking a significant step towards truly sustainable architecture.